There are five (5) major categories of compounding ingredients. They are elastomer as a major component, curatives for crosslink formation, antidegradants for protection, fillers for reinforcement and cost dilution and processing additives for ease of processing.
Elastomer is the key ingredient and thus always at the top of the formulation list. It can be a single elastomer or a blend of two or more different rubbers.
Curatives
Curatives are the main ingredients which play an important role in the the formation of crosslink structure in a vulcanized rubber. The curatives are classified as:
- Vulcanizing/curing agents such as sulphur, peroxide etc.
- Activators such as zinc oxide and stearic acid.
- Accelerators such as CBS, TMTD, MBT, DPG etc.
- Scorch retarders or prevulcanization inhibitors such as salicyclic acid, NDPA, CTP etc.
Curing agents are chemicals which impart three dimensional network or crosslink structure to the rubber molecular chains. In doing so they react with active sites in the rubber to form crosslinks between the chains.
Activators in rubber formulation are typically metal oxides (zinc oxide), and fatty acids (stearic acid). Together they form rubber soluble complexes with the accelerators making them better able to react with sulphur to develop cross-links.
Accelerators are used in small amounts to increase the rate of cure and the efficiency with which sulphur is utilised in crosslinking. Accelerators also control the onset and extent of reaction between sulphur and rubber.
Retarders are added to the rubber formulation in order to reduce the tendency of a rubber compound to vulcanize prematurely (delay the onset of cure) by increasing scorch delay.
Antidegradants
Degradation of rubber products is caused by attacks of oxygen, ozone and weathering on the rubber molecular structure. Rubber vulcanizates become brittle when they age. Aging is caused by a severe attack of oxygen and is accelerated by heat.
An antidegradant is a compounding ingredient added into a rubber compound to retard the deterioration caused by oxidation, ozone, light or combination of these. Hence antioxidants are added to protect the rubber against oxygen attack while antiozonants are added to protect the rubber against ozone attack.
Fillers
Fillers are materials that when added to a rubber formulation will either lower the compound cost or improve vulcanizate properties .
Fillers can be classified into three categories, which are as follows:
Diluents or extenders which are intended primarily to occupy space and are mainly used to lower the formulation cost. This type of filler is also called non-reinforcing filler with particle between 1,000 and 10,000 nm (1 to 10 μm).
Functional or reinforcing fillers are those with particle size range from 10 to 100 nm (0.01 to 01 μm)which when added to the rubber compound improve the modulus and failure properties (tensile strength, tear resistance and abrasion resistance) of the final vulcanizate.
In-between these two types are semi-reinforcing fillers with particle size range from 100 to 1000 nm (0.1 to 1μm).
In rubber-manufacturing industry fillers are generally classified into black (carbon black) and non-black or white fillers. These black or non-black fillers thus may come as diluents, reinforcing or semi-reinfrocing fillers depending on their particle sizes.

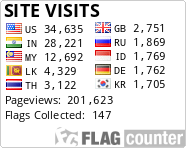